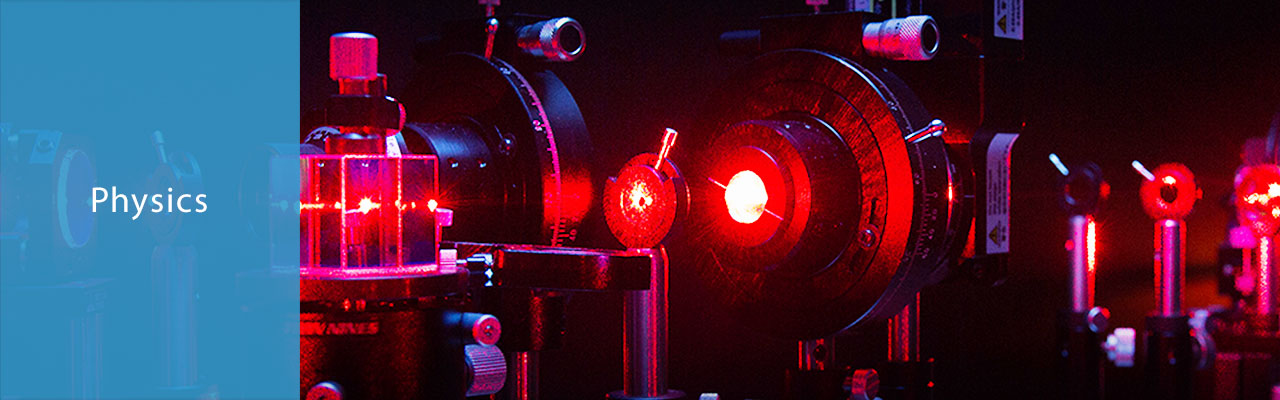
HOME / Departments / Physics / Theoretical Nuclear Physics
Theoretical Nuclear Physics
-
- OGATA Kazuyuki, Professor
- MINATO Futoshi, Associate Professor
- OGAWA Shoya, Assistant Professor
- CHAZONO Yoshiki, Assistant Professor
- The “Theoretical Nuclear Physics” research group is aiming at revealing the dynamics of many-body quantum systems, mainly atomic nuclei. Emphasis is placed on the correct interpretation of data observed in experiments. We are constantly refining our theories in order to extract new physics of many-body quantum systems with a high degree of accuracy. We are also actively engaged in discussions with theoretical and experimental researchers in the world.
What Theoretical Nuclear Physics Aims to Achieve
First, let us clarify the basic goal of theoretical nuclear physics. That is, to elucidate the nature (structure) and transformations (reactions) of composite particle systems consisting of protons and neutrons (nucleons). Some people then may have the following questions.
It is already known that protons and neutrons are made of quarks, so why do you treat them as fundamental particles? Also, since nucleon-nucleon interactions are well understood, is there any mystery in a nucleus, which is simply a collection of nucleons?
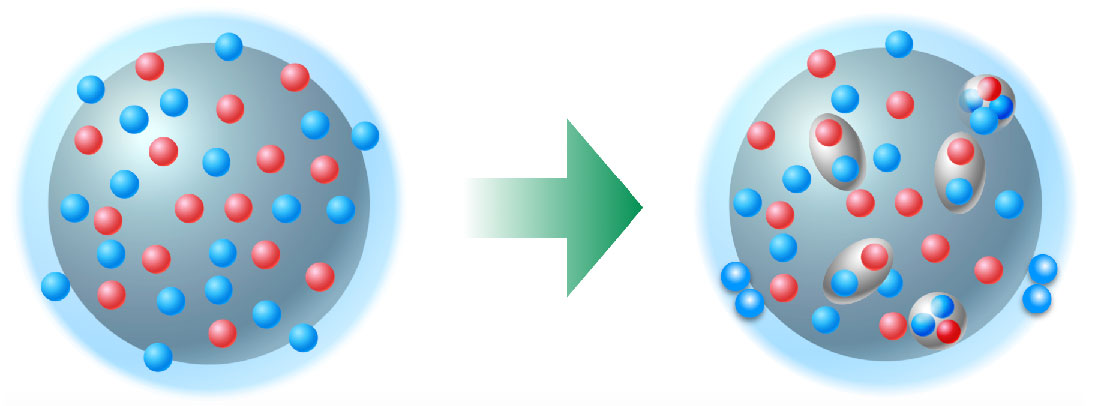
The answer is simple. Even if the basic building blocks and their basic interactions are perfectly known, we cannot predict how they will behave when they come together. For example, the existence of nuclei in the shape of bananas or hearts has been predicted, but no one knows if it is true. Also, there are theories that there are many small nuclei, like clusters of grapes, floating within the nucleus (Fig. 1). Considering these things, you can see that a nucleus is quite different from “simply a collection of nucleons.” The system of many nucleons is a very interesting subject of study because it contains very rich physics and unknown properties.
Nuclei are also relevant to our life. It is the changes in the nucleus that make the stars in the night sky shine. The elements around us were produced by reactions of the nucleus from the beginning of the universe to the present, mainly in the stars. Furthermore, the study of nuclear transmutation, that is, the process of annihilating long-lived radioactive waste in a realistic time and at a realistic cost, is also a study of nuclear reaction. The study of nuclei is connected to the physics of the universe and the physics of human society.
From our recent research
Let us now introduce some of the specific research topics that our group is working on.
1. Full picture of nuclei using the quantum “Daruma-Otoshi” reactions
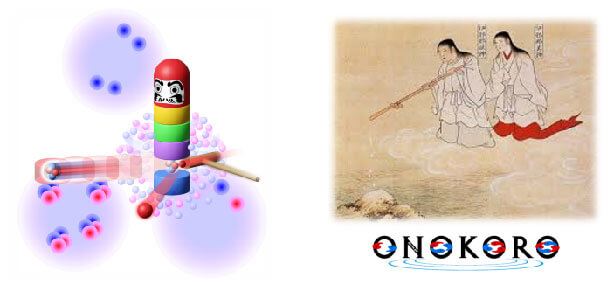
The knockout reaction has been attracting attention in recent years as a means of elucidating the rich structure of nuclei. This reaction can be likened to the “Daruma-Otoshi,” a Japanese toy shown in Fig. 2 left, in the quantum world. In those reactions, the nucleus is swung out with a “hammer” of protons of high energy, and the constituent elements of the nucleus are knocked out. The momentum distribution of the observed particles can be analyzed using quantum mechanics to determine how much of that particle was present in the target nucleus and how it was moving in the nucleus. Very recently, the first observation of an alpha particle (4He nucleus) in a middle-heavy nucleus was reported in Science 371, 260 (2021).
A series of knockout reaction studies has been named the “ONOKORO Project” and is being promoted through experimental and theoretical close collaboration. The name is taken from “ONOKORO-jima Island,” which is believed to be the birthplace of Japan in the Kojiki myth. Our group is responsible for the theoretical analysis of the knockout reaction in this project. We are particularly focused on describing the reaction that knocks out weakly-bound clusters. To what extent does striking a Daruma-Otoshi made of glass shatter the glass? And how we can extract information about the original glass parts from the shattered glass? We are currently working on these questions using quantum mechanical scattering theory.
2. The Challenge of Transmutation and Nucleosynthesis
Let us talk about the issue of radioactive waste. Disposal of nuclear waste produced by nuclear power plants, especially long-lived radioactive waste that needs to be managed for a period exceeding 100,000 years, is an extremely important issue for mankind. In response to this problem, attempts have been made to reduce the radioactive level of nuclear waste by using nuclear reactions. Recently, deuteron-based transmutation has been attracting attention (Fig. 3 left). Deuteron is relatively easy to control in accelerators because it has an electric charge, and it is easily broken up into a proton and a neutron. Because neutron is electrically neutral, it can penetrate deep into the target nucleus. Deuteron is thought to be capable of transmutation with a high probability.
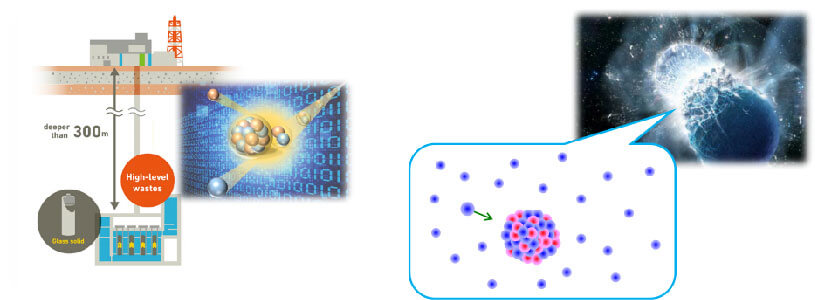
Theoretically, it is essential to describe the reaction of deuterons, including the degrees of freedom of breakup. The Continuum-Discretized Coupled-Channels method (CDCC), which was developed by the Kyushu University group, can describe this kind of breakup reaction with the highest accuracy. Currently, we are trying to construct a new nuclear reaction model based on CDCC that is applicable to reactions required for nuclear transmutation, which is the process in which a target nucleus is transformed into various nuclides. Through this research, we hope to contribute to the development of efficient nuclear transmutation technology.
Now let us talk about nucleosynthesis in the universe. It is thought that gold and uranium on the earth were produced by a process in which neutron capture and beta decay are repeated in a short period of time. Currently, this process is thought to occur at the time of neutron star mergers. Then, the following question can be raised. When a neutron star merges, there should be a large number of neutrons at that site (Fig. 3 right). What role do the surrounding neutrons play in the reaction in which the nucleus captures a neutron? We have recently found that these ambient neutrons can accelerate the reaction neutrons and significantly change the probability of elemental synthesis. We have also succeeded in systematically predicting the beta decay rate of a peculiar neutron-rich nucleus in a neutron star using machine learning. We are currently working on generalizing these results and incorporating them into cosmic elemental synthesis network calculations.
3. Physics of weak interactions occurring in atomic nuclei
Various particles from the universe are constantly raining down on the ground. For example, hundreds of trillions of neutrinos produced by the sun pass through our bodies every second. Muons, secondary cosmic rays produced when high-energy protons interact with the atmosphere, pass through the human body hundreds of times per second. What happens when such neutrinos and muons interact with atomic nuclei? The phenomena in which protons and neutrons, existing alone, react with neutrinos and muons are relatively well understood. However, because nuclei are many-body systems consisting of protons and neutrons, they behave quite differently from the reactions with single protons and neutrons. For example, imagine a baseball home run ball falling into the bleachers, and spectators near the point of impact fighting over the ball. In rattling bleachers, there is not much contact between spectators. However, if the home run ball comes flying into the crowded bleachers, contact (interaction) between spectators is likely to occur. Not only that, but you can imagine that the frequency of contact between spectators will vary depending on the nature of the home run ball, whether it is liner (fast) or parabolic (slow). In the nuclear world, such spectator behavior is regarded as the “collective motion” of nucleons and is the subject of active research. We are leading the world in this field with our research on the reactions of nucleons with leptons such as neutrinos, muons, and electrons.
4. Evolution of time hierarchy in quantum systems
Finally, let us introduce a slightly different research topic. Nuclear reactions, in fact, cover an extremely wide range of phenomena, from 10-23 to 10-16 seconds on the time scale. This time gap is equivalent to one second and one year.
Reaction processes are broadly classified into direct processes, pre-equilibrium processes, and compound nuclear processes, depending on the elapsed time (degree of reaction progress). What is interesting here is that the quantum nature of the system seems to be partially lost when going from the direct process to the pre-equilibrium process. Specifically, when we look at the angles and degrees to which emitted particles fly, we see interference stripes in the direct process, but this disappears in the pre-equilibrium process. The disappearance of the stripe pattern means that this interferential nature has been lost. Furthermore, when it comes to more advanced compound nuclear processes, the time evolution of the system is known to follow the classical Langevin equation well. We consider the change in the quantum nature of the system with the progress of the reaction (passage of time) as the “evolution of time hierarchy” and are discussing this physics with researchers in various fields.