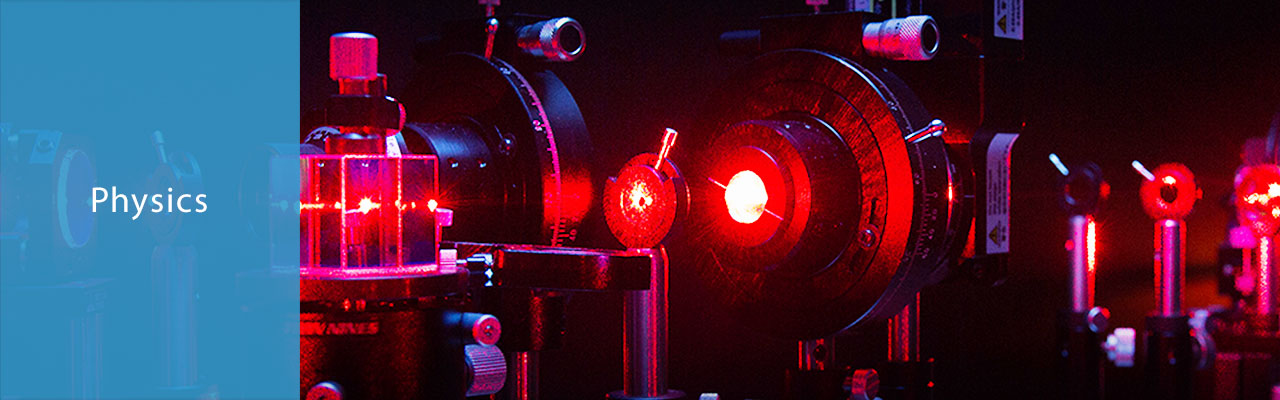
HOME / Departments / Physics / Physics of Magnetism
Physics of Magnetism
-
- MITSUDA Akihiro, Associate Professor
- Experimental study on the exotic electronic and structural properties in condensed matters by magnetic and transport measurements and diffraction and resonance techniques under high pressures; itinerant electron magnetism, valence instabilities of rare-earth elements.
Valence instability of 4f electron systems.
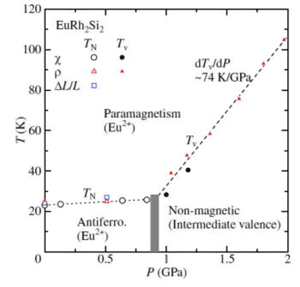
In some rare-earth compounds, 4f electrons can be delocalized due to strong mixing with conduction electrons. When the configuration 4fn of a rare earth ion is nearly degenerate with a 4fn-1 state and a surplus conduction electron, the 4f state will fluctuate between two valence states. This behavior is called valence fluctuation. The valence fluctuating state of Eu is realized between Eu3+(4f6) and Eu2+(4f7) configurations. In some cases, valence changes abruptly in its temperature dependence, which is the valence transition. Recently, we have observed that EuRh2Si2 exhibits a valence transition under pressure [1]. The temperature-pressure diagram is depicted in Figure 1. The antiferromagnetic Eu2+ state is replaced by a nonmagnetic nearly Eu3+ state at low temperatures above 1 GPa.
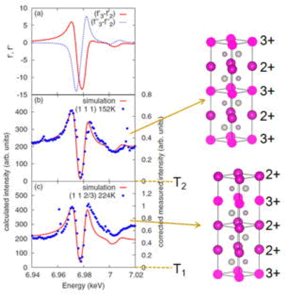
In other cases, the two valence states are ordered periodically below the characteristic temperature due to strong Coulomb repulsion between electrons. This is the valence ordering. The ternary Eu pnictide, EuPtP had been believed to be a valence ordering system. The structure contains layers of Eu and Pt-P atoms alternating in the c-direction. This compound undergoes two first-order phase transitions at T1=235 K and T2=190 K. Early reports suggested that the valence ordering takes place at T1 and another valence order state is realized below T2. We prepared single crystals and performed resonant X-ray diffraction measurements as collaboration work with JASRI [2]. The results are shown in Figure 2. We have observed (1 1 1) reflection below T2 and superlattice reflections (1 1 2/3) and (1 1 4/3) between T1 and T2. These results have revealed that the valence order patterns are Eu2+Eu3+Eu2+Eu3+ at T < T2 and Eu2+Eu2+Eu3+Eu2+Eu2+Eu3+ at T2 < T < T1.
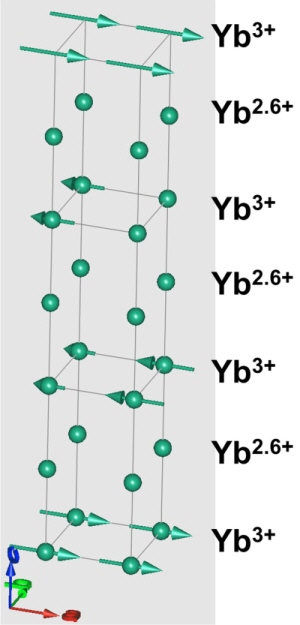
Finally, YbPd, which crystallizes in the cubic CsCl-type structure at room temperature and has four phase transitions, had also been anticipated to exhibit valence ordering. We have clarified by single crystal X-ray diffraction that YbPd exhibits a structural phase transition from cubic to tetragonal symmetry at T1=130 K and valence ordering transition at T2=110 K [3]. The valence ordering is exotic because integral valence (Yb3+) and valence fluctuation (Yb2.6+) are arranged alternately along the c-axis. In addition, Using pure powdered samples and BL20 (iMATERIA) at J-PARC, the magnetic structure below T3=1.9 K was examined by powder neutron diffraction. It was manifested that the only Yb3+ ions exhibit an incommensurate sinusoidal magnetic structure with k = (0.080 0 0.32) and an amplitude of 0.3μB [4]. The amplitude is smaller than the theoretical magnetic moment of Yb3+, which suggests that Kondo effect affects the valence ordering and destabilizes the magnetic ordering.
References
[1] A. Mitsuda et al., J. Phys. Soc. Jpn. vol. 81 (2012) 023709.
[2] T. Inami et al., Phys. Rev. B vol. 82 (2010) 195133.
[3] A. Mitsuda et al., J. Phys. Soc. Jpn. vol. 82 (2013) 084712.
[4] K. Oyama et al., J. Phys. Soc. Jpn. vol. 87 (2018) 114705.