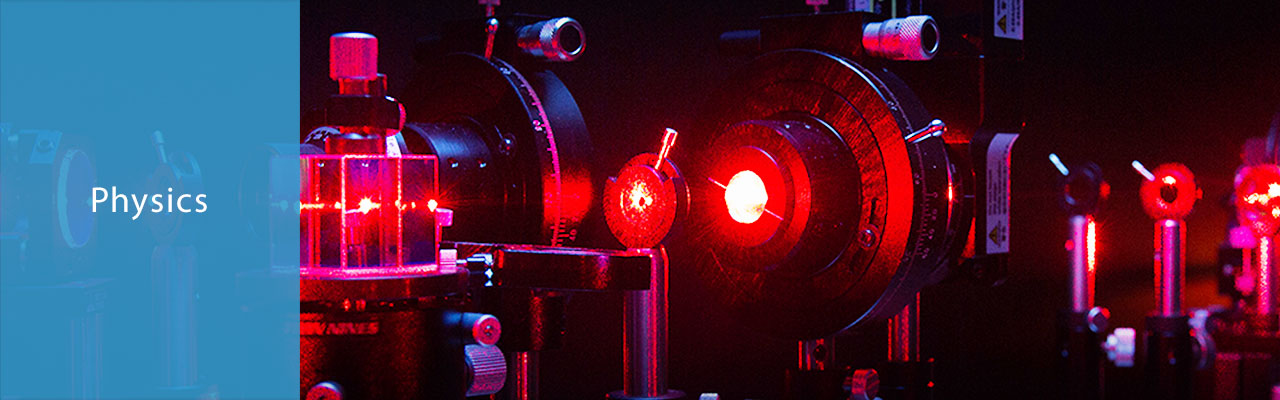
HOME / Departments / Physics / Theoretical Quantum Physics, Gravitation and Cosmology
Theoretical Quantum Physics, Gravitation and Cosmology
-
- YAMAMOTO Kazuhiro, Professor
- KANNO Sugumi, Associate Professor
- MATSUMURA Akira, Assistant Professor
- TAIRA Takano, Assistant Professor
- Theoretical studies of cosmology, gravitation, and quantum information science (cosmology and gravitation using quantum information science, quantum entanglement and quantum nature of gravity, quantum field theory of curved spacetime , cosmology, inflationary universe, gravitational waves, Tests of gravity and dark energy models with the large-scale structure of the universe)