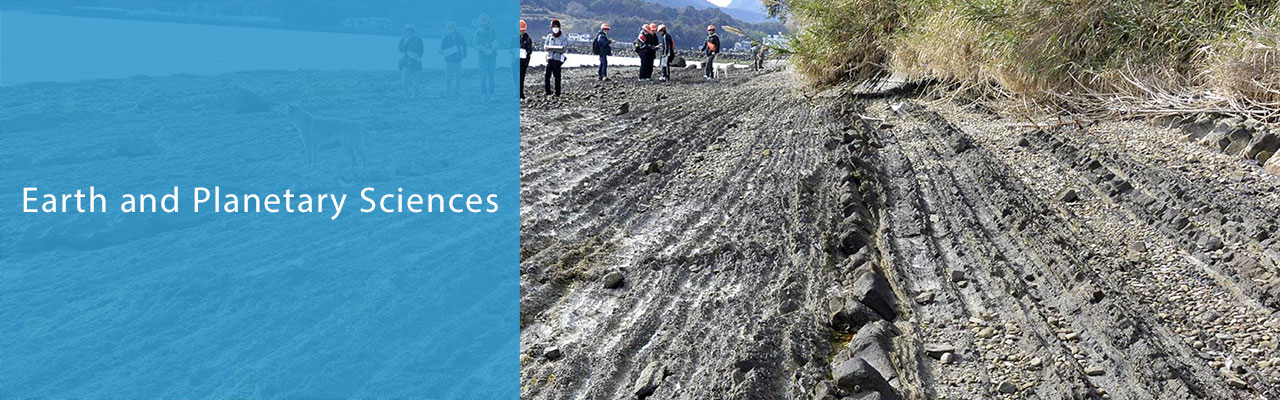
HOME / Departments / Earth and Planetary Sciences / Dynamics of the Earth's Interior
Dynamics of the Earth's Interior
-
- YOSHIDA Shigeo, Associate Professor
- We investigate fluid dynamical processes inside the Earth through theoretical studies and data analyses. The main topic is core dynamics, including geomagnetic field variations, basic dynamo processes, flow in the inner core, and the formation of the Earth's core. Other research areas include dynamics of volcanic eruptions and hydrothermal circulation.
Dynamics in the outer core
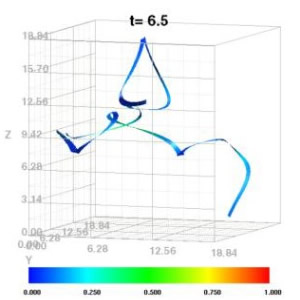
We investigate basic fluid dynamical processes in the outer core. The Earth's outer core is the origin of the geomagnetic field. (1) Part of the variations of the geomagnetic field may be a manifestation of magnetohydrodynamic (MHD) waves. We examine characteristics of MHD waves, including wave speeds and dispersion relations, and compare them with observations. In particular, we are now interested in the MAC wave confined in the stratified layer in the uppermost outer core. (2) Dynamo processes may be classified into the alpha effect and the omega effect. We study the basic mechanism of the alpha effect, which is the twisting of the magnetic field by helical fluid motions (Fig.1). We have found a non-locality in the alpha effect. (3) Thermal interaction between the mantle and the outer core may be responsible for persistent non-dipole spatial patterns of the geomagnetic field. We investigate how thermal heterogeneities on the core-mantle boundary affect the geomagnetic field.
Dynamics in the inner core
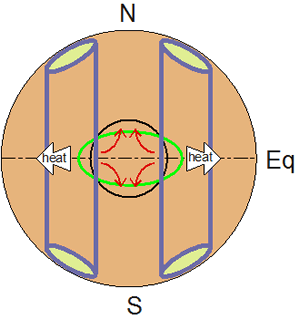
The inner core shows seismic anisotropy, with the P-wave velocity faster in the polar directions. This anisotropy is interpreted as a consequence of preferential alignment of iron crystals, which in turn results from a flow in the inner core. We model such flows in relation to the outer core dynamics (Fig.2).
Fluid dynamics of volcanic eruptions and hydrothermal circulation
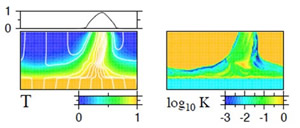
We study fluid dynamics related to geothermal phenomena, including volcanic eruptions and hydrothermal circulations. These systems exhibit interesting interplay among flow, phase change, and other thermodynamical changes. (1) Volcanic eruptions show periodic temporal changes, which may be due to an instability of a bubbly flow in the volcanic conduit. We study such instabilities. (2) In hydrothermal circulations, water flow induces chemical precipitation, which affects the pattern of a flow field. We study such interplays by numerical simulations (Fig.3).