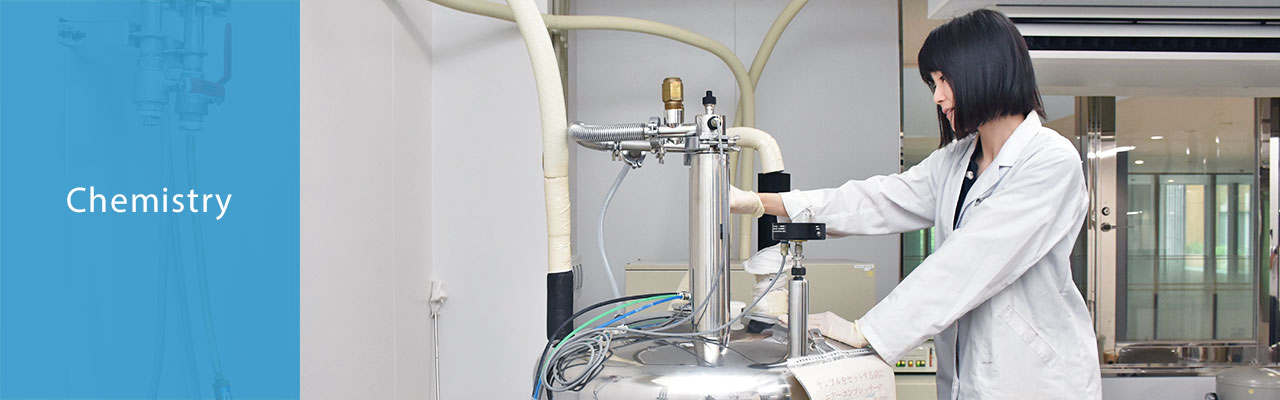
HOME / Departments / Chemistry / Quantum Chemistry
Quantum Chemistry
-
- TERASAKI Akira, Professor
- HORIO Takuya, Associate Professor
- IWASHITA Tomoya, Assistant Professor
- Lushchikova Olga, Assistant Professor
- Atomic and molecular clusters offer a unique opportunity to elucidate how physical and chemical properties of a matter emerge as atoms and molecules associate together one by one. We are interested, for example, in how ferromagnetism emerges for iron, cobalt, and nickel among 3d transition metals, whereas other elements do not exhibit it. For another example, silver nanoparticles are known to show strong photoabsorption, so-called surface-plasmon resonance, which is technologically important in coupling light with materials. However, one does not know how this picture works for small particles less than 1 nm in diameter. Furthermore, clusters provide a model of catalysts to gain molecular-level insights into mechanism of chemical reactions. We tackle these problems in materials science with single-atom precision toward advanced nano-science and technology.
1. Spectroscopy and chemical probe of size-selected clusters
Our state-of-the-art experimental technique is based on mass spectrometry enabling size-selection of clusters in single-atom precision. Figure 1 shows one of the experimental setups in our laboratory. Metal cluster ions are produced in a magnetron-sputtering cluster ion source, guided by ion guides and deflectors, and size-selected by a quadrupole mass filter. Cluster ions thus size-selected are stored in an ion trap; this is the key part of our technique to accumulate dilute sample clusters in a high density for high S/N measurement. Optionally, the stored ions are cooled down either by liquid N2 or by liquid He, and are applied with a magnetic field.
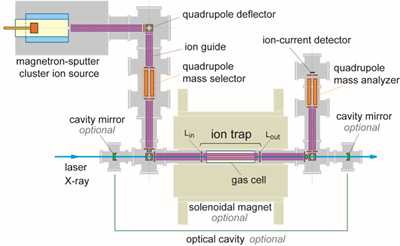
The stored size-selected cluster ions are interrogated either by laser or by X-ray spectroscopy. Laser spectroscopy probes valence electrons to measure optical absorption and magneto-optical effects. The optical responses are measured via photofragmentation and cavity-enhanced methods. X-ray probes inner-shell electrons: X-ray absorption spectroscopy (XAS) provides chemical analysis of constituent atoms and X-ray magnetic circular dichroism (XMCD) reveals magnetism. The cluster ions are interrogated by foreign molecules introduced into the ion trap as well. Chemical reactivity and reaction pathways are measured by employing a mass analyzer for product analysis.
2. Study of chemical-reaction pathways step by step
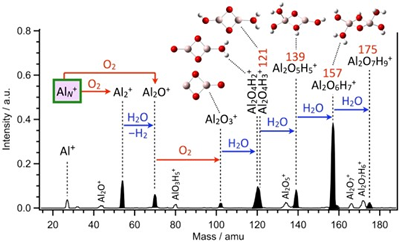
One of the advantages of our experimental technique is that we are able to elucidate elementary processes of sequential chemical reactions step by step. We have demonstrated this for formation of hydrogenated hydrated-alumina cluster ions, Al2O3(H2O)NH+, from aluminum cluster ions, AlN+, in the presence of oxygen and water molecules representing a natural atmospheric environment. Figure 2 shows a mass spectrum of product ions upon reaction of AlN+ with O2 and H2O. We were able to disentangle the reaction pathway by means of step-by-step experiment as follows:

The predominant products at 139, 157, and 175 amu were identified as Al2O4H3(H2O)1+, Al2O4H3(H2O)2+, and Al2O4H3(H2O)3+ with one, two, and three H2O molecules remaining intact, respectively, with the aid of collision-induced dissociation experiment to obtain further structural information. The chemical composition of these products is similar to that of bauxite, which is a form of bulk aluminum abundant naturally. This study thus models oxidation, hydroxylation, and hydration steps on an aluminum surface in natural environments. It was revealed that reactions with O2 and H2O to form alumina, Al2O3, at initial steps are followed by successive hydroxylation and hydration reactions.