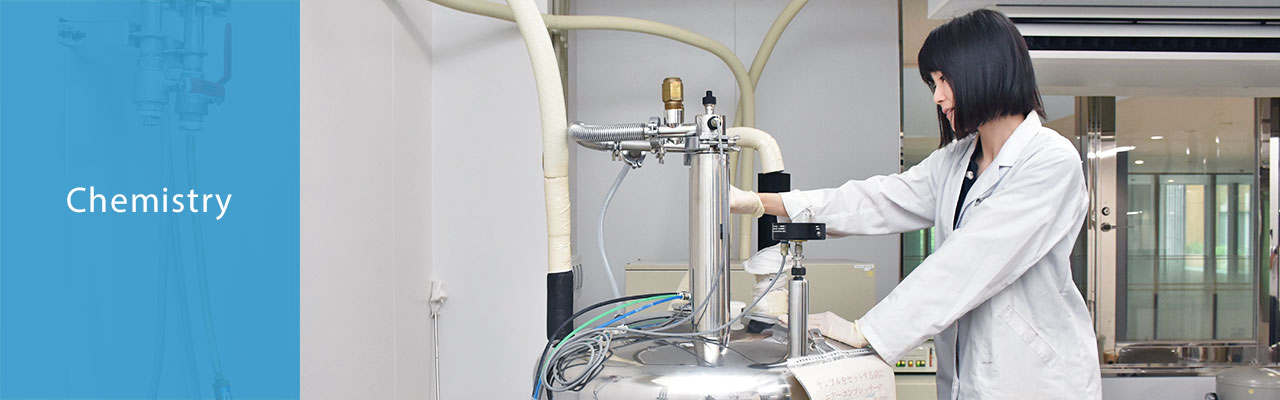
HOME / Departments / Chemistry / Physical Chemistry of Soft Matters
Physical Chemistry of Soft Matters
-
- ANNAKA Masahiko, Professor
- MAKI Yasuyuki, Associate Professor
- YASHIMA Shintaro, Assistant Professor
- We study the physical chemistry of soft condensed matter, which are easily deformable by external stresses, electric or magnetic fields, or even by thermal fluctuations. These materials typically possess structures which are much larger than atomic or molecular scales; the structure and dynamics at mesoscopic scales determine the physical properties of these materials. The goal of our research is to probe and understand this relationship. We study both synthetic and biological materials; our interests extend from fundamental physics to technological applications, from basic materials questions to specific biological problems.
1. Bio-related hydrogels
This research focuses on the properties of common soft matters. We study colloids, polymers and gels. Our focus is on understanding the structure of these materials and how this controls their dynamics and properties. We make extensive use of the experimental tools, including optical microscopy, light scattering and rheology. We also invent new tools and experimental techniques to explore all properties of the material. Much of our focus is on the mechanical properties of the materials and their relationship to the internal dynamics of the structures within the material.
Hydrogels are an exciting platform technology for solutions in many high impact areas, including injectable biomaterials for tissue reinforcement, and extensible networks for applications requiring flexibility. Precision engineering of hydrogels is essential to developing high performance materials such as these, which also need to be adapted to a variety of practical processing conditions. Our efforts are focused on developing well-defined macromolecular systems that can be used to study the fundamentals physics of gels at the nano-scale, develop new mechanisms of responsive gel reinforcement and toughening, and engineer bioactive materials for controlled cell activity, hemostasis, and tissue integration and adhesion. Control over gel structure allows us to investigate the structure-property relationships that are critical determinants of gel performance, including the role of network topology on the mechanics of chemical and physical gels, as well as the role of nanostructure morphology on the mechanics of supramolecular gels. In addition, we are developing hierarchically structured gels that can capture the complex viscoelastic behavior of natural tissues.
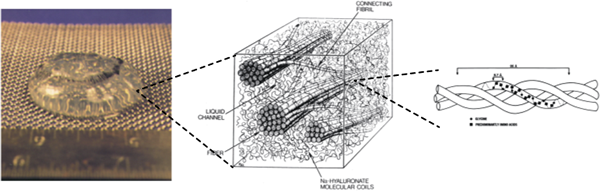
2. Biophysics
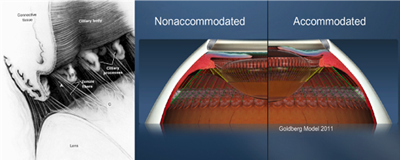
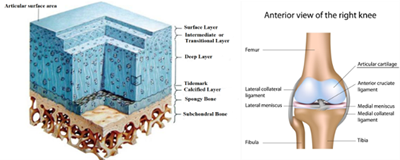
We use our expertise and our experimental tools to investigate the properties of biological materials. Much of our focus is on developing an understanding of the mechanical properties of biopolymer networks, formed by reconstituting proteins into gelled networks. These include cornea, lens and vitreous body in the eye, and cartilage, a flexible connective tissue, including joints between bones. We combine visualization of the network structure with probes of their mechanical properties to understand the nature of these properties. In addition, we extend our investigations to study the mechanical properties of cells and collections of cells.
3. Friction of hydrogels
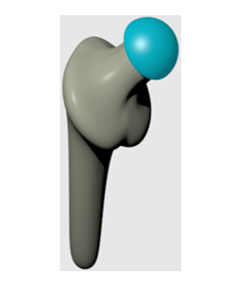
We usually do not feel any friction reduction when our eyes and joints are moving. The smooth motion of bio-tissue is due to the low friction between eye and eyelid, and articular cartilages. More examples can be observed in blood transportation in which red blood cells deform and smoothly squeeze through capillaries with a substantially smaller diameter than their own, owing to the low friction between the red blood cells and the capillary wall. In order to understand the low friction mechanism of bio-tissue, the friction behavior of hydrogels are drawing the attention around the world, because of its soft and wet properties. We, thus far, especially focus on the effect of surface geometry on hydrogels friction. Surface geometry is the key issue in any friction event, and is expected to control the friction of hydrogels. One of our goals through the research on hydrogels friction is to acquire the knowledge to produce an artificial joint cartilage made of hydrogels, which may show better biocompatibility than the present one which made of hard materials (metal or HPPE).
4. A new biomedical imaging technology
The goal of this project is to develop and validate a new biomedical imaging technology based on Brillouin light scattering for non-invasively measuring the biomechanical and rheological properties of tissues, cells, and biomaterials. Spontaneous Brillouin scattering arises from the interaction of light and sound wave that is inherently present in a sample. By detecting the spectral shifts in the scattered light, the sample’s hypersonic viscoelastic properties can be measured at microscopic spatial resolution without physical contact. Brillouin spectroscopy has long been known in material physics and environmental sensing. However, its potential applications in biological sciences and clinical medicine have not been fully explored. We aim to develop new practical instruments and validate them for a number of potential applications. Brillouin imaging has a potential to be a useful diagnostic tool in ophthalmology for early detection and screening of various lens and corneal problems, such as presbyopia, cataracts, and corneal ectasia. This technology may also be useful in the study of cellular biomechanics in cancer and tissue engineering.