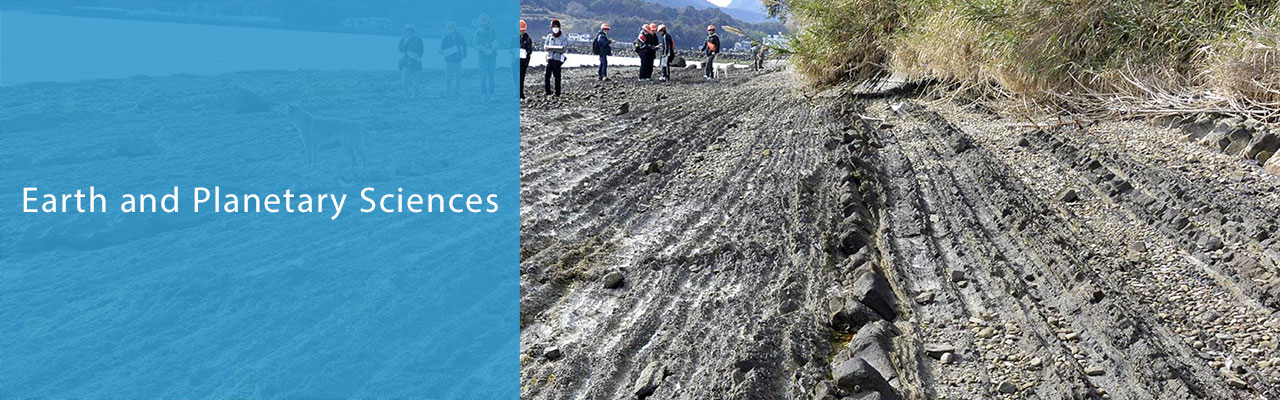
HOME / Departments / Earth and Planetary Sciences / Deep Earth Materials Science
Deep Earth Materials Science
-
- KUBO Tomoaki, Professor
- TSUBOKAWA Yumiko, Assistant Professor
- Studies on the properties of the Earth and planetary constituent minerals provide the certain proof of the elementary process operated in the evolution of the planets. Our group focus the dynamic properties (rheology, faulting, diffusion and reaction kinetics) under high pressure. Multi-anvil system and diamond anvil cell are used to generate the high pressure and temperature conditions of the planetary interiors. Synchrotron X-ray sources at Spring-8 and KEK are essential to in-situ observation method. The former and future research topics are given below.
1. Phase transition and rheology of deep Earth materials
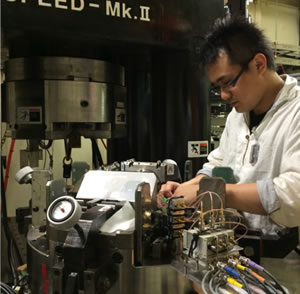
High-pressure phase transition caused internal stratified structure of the terrestrial and icy planetary bodies. The heat and material transport by solid state convection in such regime controls characters of the surface tectonics. In case of the Earth, subduction of the cold plate into the mantle carries substantial amounts of water and the components with low melting temperature to the deep layers. The volcanic and seismic activities in the deep Earth origin are related to dehydration, melting, and phase transitions of the minerals in the subducting plate. Especially, we try to solve the problems; On what conditions subducted plate could penetrate or be stagnated at the upper and lower mantle boundary? Why deep earthquakes occur beyond brittle–ductile transition exclusively inside the plate? How does convective mixing of the chemically differentiated plate occur in the lower mantle and D″ layer? By the experimental approach consisting of plastic deformation and acoustic emission measurement, we study coupling phenomena of phase transition and rheology at high pressure (Fig. 1).
2. Shock metamorphism in meteorites
We also study formation process of high-pressure minerals in shocked meteorites to investigate collisional history of asteroids and formation process of planets in early sola system. In general, short period of impact caused metastable transition of minerals and non-equilibrium texture, which are preserved in shocked meteorites. Reproduction of such characters by high-pressure experiments (Fig. 2) enables us to evaluate the impact conditions (pressure, temperature, and duration and consequently impact velocity and size of impactor).
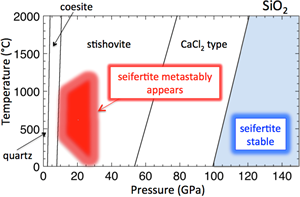
3. Rheology of planetary ices
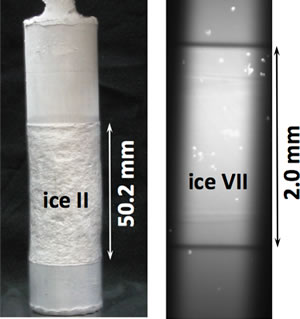
Various types of icy moons have been found in the outer solar system. Icy super-Earths have also been detected as exoplanets. Thermal convection in ice shells and mantles of these icy bodies is critical to understanding their thermal histories, internal dynamics, surface tectonic activities, and survival of internal oceans. Ice viscosity is one of the most important parameters for occurrence and style of internal convection. We experimentally investigate rheology, polycrystalline kinetics, and diffusion of planetary ices such as water ice, high-pressure ices, and non-water ices (Fig. 3). They are shown to unexpectedly be weak under low-stress condition in the interior of icy bodies, and aggregation with small amount of CO2 ice has a profound effect to further decrease the strength and viscosity. These results can be used to constrain tectonic activities and internal convection of icy moons and planets in extremely low temperature environment.