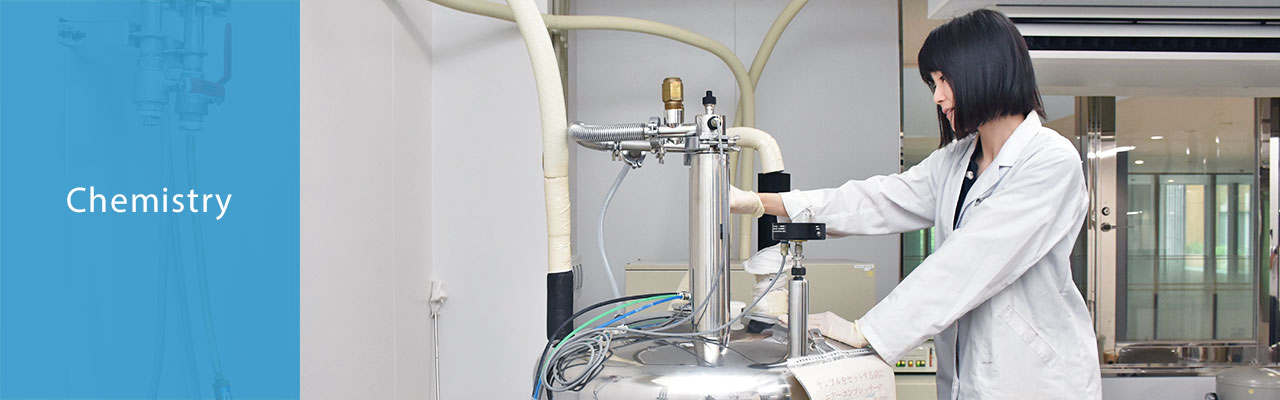
HOME / Departments / Chemistry / Chemical Physics and Biophysics
Chemical Physics and Biophysics
-
- AKIYAMA Ryo, Associate Professor
- Various chemical and biological phenomena, such as molecular motor, proteins’ association, and electron transfer reaction, are studied on the basis of physics in our group. We are interested in medium effects, such as solvent effects. Those effects have been recognized to be minor. However, media often change the fates of solutes drastically. Actually, proteins work only under certain thermodynamic condition, and stabilities of colloidal dispersion systems are regulated by the system temperature, the salt concentration, and so on. And, those phenomena relate to the biological functions. We are basically interested in those phenomena. Our main tools are thermodynamics, statistical mechanics and numerical calculation by using computers, such as molecular simulations.
1. Meetings
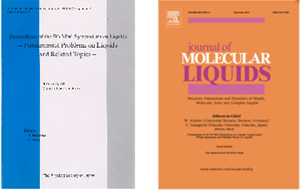
Every summer our group provide a meeting to discuss liquid matters with Koga group in Okayama University. The series of meetings are Mini-Symposium on Liquids (MSL). Two special issues have been published from Journal of the Physical Society of Japan and Journal of Molecular Liquids (J. Phys. Soc. Jpn. Supplement A 81(2012), J. Mol. Liq. 200A (2014), Fig.1). And we often have open seminars.
2. Educations in our group
Basis set of knowledge in our group is analytical mechanics, namely Lagrangian and Hamiltonian mechanics, and statistical mechanics. The students in our group must learn them. To master them we prepare the tutorials of exercises and discussions by using some text books every year. And the students must learn FORTRAN programing. The goal of the subject for computation is construction of molecular dynamics simulation for Lennard-Jones particles.
3. Studies
We are interested in liquid matters related to biological phenomena, especially medium effects (Fig. 2).
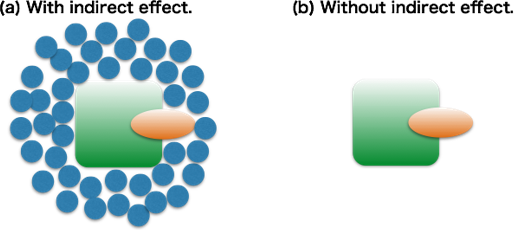
3.1 Attraction between negatively charged macromolecules
Like-charged particles are repel each other in vacuum. However, attraction between like-charged particles is observed in biological systems. For example, actin monomers attract each other in biological systems and construct fibrils, although they are negatively charged proteins.
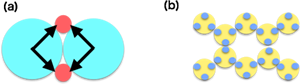
We calculate the effective interaction between macroanions immersed in an electrolyte solution by using an integral equation theory, which is based on statistical mechanics. The theoretical results indicate the attractive interaction between macroanions. The attraction is mediated by small cations (Fig. 3a). Interestingly, the effective attraction appears under certain electrolyte concentration. So, those macroanions are repel each other in dilute electrolyte concentration, and the effective attraction appears when the concentration is mM order. However, the attraction disappears if the concentration increases. This reentrant behavior of the attraction is also observed in the experimental results for acidic proteins condensation, and DNA compaction. We are studying the phase behaviors of aggregation and the application for molecular motors (Fig. 3b).
3.2 Effective attraction driven by translational motion of solvent molecules
Is entropy an index of randomness? The answer is NO, we think. Actually, there are so many experimental results of molecular recognition processes driven by entropy gain. Another famous example is Alder transition: the system’s entropy becomes larger in the crystallization process of hard spheres under the isochoric condition. Thus, self assembly processes often increase the system entropy.
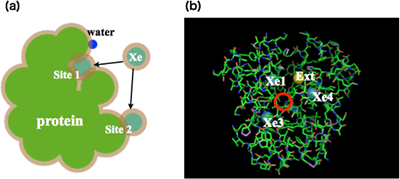
Asakura–Oosawa theory is one of the simplest theory to understand those phenomena. When Asakura and Oosawa proposed this entropic attraction between two spherical bodies in 1954, the spherical bodies were immersed in a dilute macromolecular (or polymer) solution and the attraction between the spherical bodies was driven by macromolecules (polymers). This idea is so nice. However, the effects of solvent molecules were ignored and the solvent effect often becomes important. The idea of Asakura-Oosawa theory must be applied to the entropic attraction which is driven by the translational motion of solvent molecules under certain conditions. We applied this idea to the molecular recognition, such as adsorption of anesthetic molecules on Myoglobin and etc. (Fig. 4). The calculated adsorption sites and stabilities are reasonable.
We also study dielectric behaviors of aqueous solutions, diffusion behaviors of macromolecules, molecular motor, and so on.